Terrestrial cyanobacteria in Antarctica
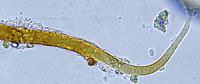
Figure 1. Ammatoidea normanii, a cyanobacteria known from both Antarctica and the New Zealand alpine zone. Image - Phil Novis
Terrrestrial cyanobacteria in Antarctica are frequently encountered in lichens: at least 13 species of Antarctic lichen fungi associate with cyanobacteria as the main photosynthetic partner, and a further five species incorporate cyanobacteria in their cephalodia. However, many cyanobacteria are quite hardy enough to survive in Antarctic terrestrial ecosystems without any assistance from lichen fungi.
Free-living filamentous cyanobacteria have been found on nunataks in the La Gorce Mountains, not far from the South Pole. (As an interesting aside, the same species has been found in New Zealand alpine soils; Figure 1.)
Some, such as Nostoc commune, may even dominate ecosystems in their free-living form. In addition to its ability to fix carbon and nitrogen, Nostoc can produce masses of jelly-like mucilage that protects its cells from both desiccation and freezing (Figure 2). This combination of properties gives Nostoc a tremendous advantage in the harshest Antarctic environments, where nutrients are low, water supply is very unpredictable and many freeze–thaw cycles per day are common. Some populations are found on soil, periodically irrigated by melting snow and ice. Other populations are submerged more-or-less permanently, and are usually buffered from the air beneath an ice layer covering the pond that they inhabit. The ecology of Nostoc, therefore, can be quite variable.
![]() |
Figure 2. Colonies of Nostoc arrayed on trays for measurement of photosynthesis in the laboratory (upper). The magnified views (x400) below show the effect of different growing conditions – limited ability to produce mucilage results in tightly (left) versus loosely (right) packed filaments. |
The evolutionary implications of this variability may be profound. Populations of Nostoc surveyed from throughout Victoria Land – from latitudes as far south as the Miers catchment and as far north as Cape Hallett – demonstrate that patterns of genetic diversity can be explained by type of habitat. A technique called AFLP – a method of DNA fingerprinting – was used to compare genotypes across collection sites. Briefly, the DNA from a Nostoc sample is purified by grinding up the colony and removing the lipids, pigments and other contaminants. The DNA is then digested with a pair of enzymes that cut the molecule only at specific sites (particular sequences 4 and 6 base pairs long). This leaves a solution of DNA fragments of various sizes; some of these can be selectively amplified by attaching adaptor sequences to the fragment ends and using them as a template to polymerise more DNA. When the solution is run out on a gel to separate the fragments of different sizes, each sample gives a characteristic pattern of bands that can be compared with other samples, resulting in a measure of similarity. The phylogenetic tree shown in Figure 3 was constructed using these data.
Figure 3 shows that the Nostoc colonies collected from ponds are more closely related to each other than to those collected from irrigated soil. This is the case independent of latitude: for instance, samples from irrigated soil at Cape Hallett and Taylor Valley group together even though they are far apart geographically. The easiest explanation for this is that particular genotypes have been selected from an available pool under the constraints imposed by particular habitats.
![]() |
Figure 3. A splitsgraph created using AFLP data from populations of Nostoc throughout Victoria Land. The populations have evolved in a tree-like fashion, and are split according to habitat. |
What are these constraints? An obvious ecological function to test is photosynthesis, the process described in the article on cyanobacteria. It was already established that the productivity of cyanobacterial populations beneath an ice cover is driven by irradiance. Because the water is thermally stratified beneath the ice and buffered from the rapid changes in temperature above, light has the greatest effect on productivity of the population. But what about populations exposed on the soil?
For them, it turns out that temperature regime is most important. This can be seen by measuring the photosynthetic response of terrestrial Nostoc to changes in irradiance and temperature in the laboratory, and determining their mathematical relationships. These models can then be used to estimate annual productivity based on real temperature and irradiance data collected by weather stations over years in the field. Although a graph of annual productivity against irradiance in terrestrial populations shows no patterns, using temperature instead gives a convincing straight line (Figure 4). Recall that in submerged populations, the reverse is the case. Here, then, is a possible local environmental stimulus that has led to the genetic divergence between soil and pond populations of Nostoc throughout Victoria Land.
![]() |
Figure 4. The modelled relationship between temperature and annual productivity in terrestrial Nostoc from the McMurdo Dry Valleys. |