Start and stop rules in pest eradication
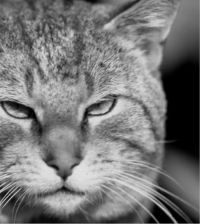
Island Conservation has been attempting to eradicate feral cats from San Nicolas Island in California.
Eradication is usually the preferred option to manage pests because it (a) eliminates any future impacts, (b) may allow the damage from past impacts to ameliorate, and (c) does so at a one-offcost. However, judging whether eradication is possible is often not straightforward. Managers can use two approaches to see whether eradication is feasible. Firstly, they can look at precedents: who has succeeded before against this pest under similar circumstances? Secondly, they can analyse whether the necessary conditions for success can be met: can all the pests be put at risk and killed faster than they can replace their losses, is immigration unlikely or manageable, do the benefits outweigh the costs, and is eradication socially acceptable?
Having decided that eradication is feasible, managers have two strategies they can use, depending on the pest species of concern and the control tools available. For some pests, eradication can be achieved by a single, one-hit control event that kills the entire population, e.g. aerial baiting of rodents on islands. For others, eradication is achieved by successive control events that eventually reduce the population to zero, e.g. ground-based hunting of ungulates or trapping of stoats. These two strategies force managers into two quite distinct decision points that determine different planning and research needs – when to start and when to stop.
For the first strategy, everything must go right ‘on the day’, so meticulous planning and review before the operation are best practice. That is, start rules are the key. The operation itself provides no (or at best limited) information on success or failure. For managers to measure this they have to check for survivors, and if any exist they have to be located and removed. However, there are problems with doing this immediately after an aerial operation – absence of evidence does not mean absence of survivors and the only way to increase certainty of success is to diligently look for survivors throughout the entire operational area. A second problem is that the detection of a survivor at one point does not provide information on their presence or absence at other places, and unless the response to a point location is to repeat the broad-scale control everywhere as a precaution, the only other way to check for ‘success’ is again to look everywhere. Both these options are costly so the normal process is to wait and see if pests have survived by allowing time for their population to recover and become obvious – and then repeat the eradication attempt. The problem with the absence of information provided by the control method per se is that the causes of failure are often difficult to prove and managers have to rely on weight-of-evidence diagnoses.
Recently, several pest eradication projects based on aerial poisoning have either not met their start rules, or have failed despite concerns about aspects of their start rules. For example, the rodent and rabbit eradication on Australia’s subantarctic Macquarie Island planned for 2010 was halted (and hopefully just postponed until 2011) as bad weather precluded the helicopters used to sow the poison bait from flying during the window of time when non-target birds at risk from the baiting were absent. In contrast, the kiore eradication on Hawaii’s Lehua Island had reached such a stage of planning (and regulatory commitment) that it was attempted and failed despite less than ideal conditions (unexpected rainfall causing a flush of vegetation and abundant food for the rats) and constraints (restrictions on baiting along the coast).
The second strategy, successive removal events, provides managers with information from the control events. Control effort, numbers of animals removed, their location, sex, age, and reproductive condition can all be measured as the campaign proceeds. Such information allows managers to adapt their plans and improve their chances of eventual operational success. The problem is thus not the start rules but when to stop and declare success.
In recent years Dave Ramsey, John Parkes and their co-workers have been using Bayesian modellingapproaches (which allow for the generation of statistical likelihoods based on partial information) with data collected during such projects to (a) measure the probability that the lack of further animals killed or caught by the control tools (or detected in monitoring devices) equals no animals present, and (b) to prescribe how much more monitoring is required and where it should be applied, to increase this probability to some level of comfort to decision-makers so they can stop all control.
For example, Island Conservation, a California-based NGO, has been attempting to eradicate feral cats from San Nicolas Island in California. Most cats were trapped over a year and this, along with searches of cat sign and the use of fixed cameras, provided data to calculate whether eradication had been achieved once no further cats were detected. Analysis of the data towards the end of the campaign suggested there was a 95% chance that between one and four cats remained, and as it transpired two further cats were detected and removed. The model that predicted this two-cat outcome had a 25% chance of being correct. The model allowed managers to design their stop-rule monitoring to limit the chance of falsely declaring eradication and/or to optimise the trade-offs between the costs of extra monitoring and the costs of falsely declaring success (see Ramsey et al. in the publication list).
A research area of growing interest is whether the approaches used on San Nicolas Island can be applied in a cost effective way to locate and kill survivors immediately after aerial eradication attempts.
This work was funded by The Nature Conservancy and Island Conservation (USA).